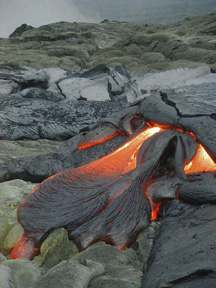 |
When the lava cools and hardens (inset), its isotopic compositions reveal much about mantle plume structures and magmatic processes.
|
The CIG was established by Berkeley Lab's Earth Sciences Division in collaboration with the University of California at Berkeley. Scientists here study the geological history of our planet and test the computer models and simulations of their colleagues through the study of radioactive and stable isotopes.
Isotopes are atoms whose nuclei contain identical numbers of protons but different numbers of neutrons. A radioactive isotope is unstable; through the emission of radiation, it will break down or "decay" until it becomes a specific stable (non-radioactive) atom. The average rate at which this decay takes place for a given type of "radioisotope" is characterized by its "half-life"-the time it takes for half of the atoms of the isotope in a sample to decay into a stable product.
Since half-lives never change, scientists can use the ratio of a specific radioisotope to the stable isotope into which it decays as a sort of geological stopwatch. Measuring the concentrations of select radioactive and stable isotopes in rock or mineral samples, then comparing the ratios between them, enables scientists to calculate when the sample was formed. They will also find clues as to how the sample was formed.
Isotope concentrations are measured through mass spectrometry. Typically, a microsample of rock is dissolved in acid and elements of interest are separated via gas chromatography. A miniscule amount of a single element, maybe no more than one ten-millionth of a gram, is deposited onto a special filament, inserted into a mass spectrometer, and vaporized. The constituent isotopes within that element are then ionized (given an electrical charge) and focused into a beam which is directed through a magnetic field. The isotopes are sorted according to mass by the magnetic field and directed into detectors where their identity and concentrations can be determined.
A Little of Everything
in All Things
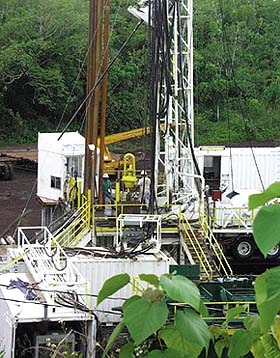 | |
In the Hawaii Scientific Drilling Project, scientists are core-drilling into a nearly extinct volcano on the island of Hawaii and removing samples of ancient lava rock. These samples are then analyzed for changes in isotopic compositions that took place over the volcano's active life.
|
"Everything in the periodic table can be found in all things, even though the quantities may be very tiny," says DePaolo. "If we can vaporize a sample and focus its ions, we can analyze its isotope concentrations."
CIG researchers have been using "thermal ionization" to prep their isotopes for mass-spectrometry. The high temperatures involved create ion beam focusing problems that limit the application of thermal ionization to only about a third of the elements on the periodic table. To overcome this limitation, the CIG is adding a new mass spectrometer that features a plasma torch and a hexapole magnet which can vaporize any element and focus the ions into the required beam.
"This machine will enable us to vaporize and ionize a sample directly without the need for dissolving and depositing portions of it onto a filament," says DePaolo. "We expect it to expand our capabilities in isotope geochemistry much like the Advanced Light Source expanded capabilities for working with x-rays."
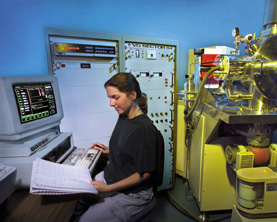 | |
Here research associate Helene Tolliver runs samples on a mass spectrometer located on the UC Berkeley campus.
|
With practically the entire periodic table now open to them, the isotope ratios that the CIG researchers study will depend upon the increment of time they wish to measure. For example, the most widely used of all radioisotopes is carbon-14, which decays to the stable nitrogen-14. With a half-life of 5,770 years, carbon-14 is ideal for dating biological materials and human artifacts, but not for tracking continental drift.
Geological dating calls for measuring much longer-lived radioisotopes, such as uranium-235 and uranium-238, with respective half-lives of 700 million years and 4.5 billion years (the age of Earth's oldest rocks). CIG researchers have been focusing on isotopes that can fill in the gaps between these two staples. With the right choice, they can measure the incremental creep of tectonic plates, determine the chronology of ice ages, or assess the formation of rocks in the earth's crust and those coughed up from the mantle during volcanic activity. They can even study meteorites that predate the earth.
In addition to his director duties, DePaolo also oversees the CIG's radioisotope work. Among his recent projects has been the potential use of isotopes for predicting the severity of volcanic eruptions. This arose from a discovery he helped make in 1993 that showed a strong correlation between neodymium isotope compositions and the volume of lava that an eruption produces. The discovery was made at Mount Unzen, a volcano in Japan with a history of eruptions dating back some 300,000 years.
"Our results suggested that neodymium isotope compositions of early or precursory eruptive lava could be a qualitative indicator of the maximum size of a continuing or impending eruption and might be used in volcanic hazard evaluation," says DePaolo.
The isotopic composition findings at Mount Unzen were similar to volume-composition patterns that have been found in volcanoes across the western United States, particularly in Yellow-stone National Park and around California's Mammoth Lakes. This neodymium signature could prove to be a life-saving measure for predicting the eruption sizes of these and other volcanoes that sit atop continental-type crust.
DePaolo is also co-leader of a project to study the lava of volcanoes that sit atop the ocean floor, such as those that make up the Hawaiian island chain. Oceanic volcanoes are thought to form when a plume of hot solid rock from the deepest levels of the mantle rises underneath the ocean floor to create a "hot spot" of magma. The goal of the Hawaii Scientific Drilling Project (HSDP) is to core-drill some 4,500 meters into the flank of a large oceanic hot spot volcano and continuously sample the solidified lava that has accumulated over a period of nearly one million years.
"Isotope ratios in the sequences of lava flows from oceanic volcanoes make uniquely valuable probes of mantle plume structures and related magmatic processes," says DePaolo.
For the HSDP, DePaolo and his colleagues selected Mauna Kea, a 13,762- foot-high oceanic volcano on the big island of Hawaii made famous by the many telescopes on its peak. Nearly extinct, Mauna Kea has gone through all its major life stages, the records of which have been preserved in the solidified lava that now lies underground.
Stable Isotopes and Noble Gas
In addition to state-of-the-art instrumentation, the CIG is also distinct for being one of the few places where work is done on stable as well as radioactive isotopes. The CIG's stable isotope studies are led by Mack Conrad. Mack Kennedy heads a noble gas laboratory, Scott Mountford runs an analytical chemistry program, and Kuni Nishiizumi is responsible for a lab that prepares cosmogenic isotopes-isotopes which are produced in the continual bombardment of surface rocks by cosmic radiation.
Stable isotopes ratios play a key role in a relatively new field called "isotope diagnostics." This involves the measurement of stable isotopes in groundwater or soil gases to get a better understanding of the chemistry that is taking place. The information is then used to predict potential environmental and health concerns and to help assess possible remediation strategies. Conrad recently employed isotope diagnostics to show that water percolates through the rock at Yucca Mountain, proposed site of a permanent nuclear waste repository, much faster than existing models predict.
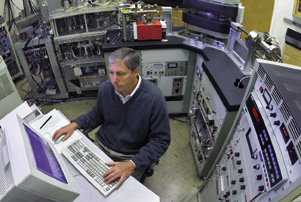 |
Mack Kennedy heads a noble gas laboratory in which it was shown that high-pressure fluids from far away are making the San Andreas fault even more prone to earthquakes.
|
We found it took water 30 years to percolate through 300 meters of soil rather than the 100,000 to one million years predicted by some numerical models," says DePaolo. "This changes the nature of the debate among hydrologists as to which model is best."
A recent noble gas study by CIG's Kennedy and Yousif Kharaka may also mean a reexamination of existing models, in this case, models that explain the presence of high-pressure fluids in fault zones. High-pressure liquids or gases can weaken faults, making them even more prone to quakes. One of the most infamous examples is the San Andreas fault in California, where the North American and Pacific plates meet.
Scientists suspect that high-pressure fluids act as lubricants, making it easier for rocks in a fault zone like the San Andreas to be displaced. However, they need to know much more about these fluids, starting with the basic question: where do they come from? Two competing models attempt an answer. One says high-pressure fluids originate in the crust, seep into the fault zone and become trapped by mineral reactions. The other says these fluids originate in the mantle and get siphoned into the fault zone through the ductile base of the crust.
To test these models, Kennedy and Kharaka measured high-pressure fluids along the San Andreas fault for ratios of helium isotopes. To their surprise, they found ratios of the exceedingly rare helium-3 (helium with only one neutron) to common helium-4 (two neutrons) that were hundreds of times higher than the helium-3 to helium-4 ratios typically found in crustal fluids.
"Some of this fluid could have come only from the mantle," says Kennedy who believes that as fluids move upward from the mantle, helium-3 becomes increasingly diluted by the helium-4 that is common to the crust. This makes it difficult to determine the degree to which high-pressure mantle fluids contribute to the weakness of the San Andreas Fault, but the effect is thought to be large. The Kennedy and Kharaka study also raises the possibility that mantle fluid is flowing into the San Andreas Fault from great distances away.
DePaolo once likened Earth to an ongoing experiment in which good notes were kept. Through isotope geochemistry, he and his CIG colleagues are continuing to find new and better ways to decipher those notes.end
|