Shank’s First Years: Fundamental Research and National Needs
A legacy of achievement: Top Quark and Relics of the Universe
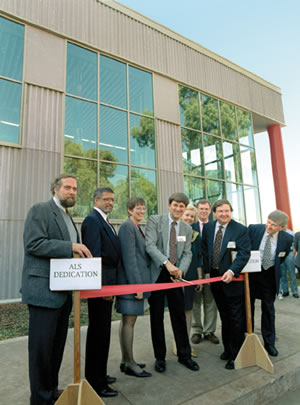
ALS dedication with (left to right) Jay Marx, Don Perlman, Martha Krebs, David Shirley, Gail Wilson (Governor’s wife) Brian Kincaid, Charles Shank (director of LBL) and Hermman Grunder.
Charles Shank became the fifth director of Berkeley Lab on September 1, 1989. He was the first director from outside the Lab, coming to the Lab after a 20-year career at AT&T Bell Laboratories, where he made pioneering contributions to the study of ultrafast laser pulses and fiber-optic communications.
His background in cutting-edge science that quickly found a home in the private sector shaped his leadership style. He viewed national labs, and Berkeley Lab in particular, as a place to conduct fundamental research and a place to incubate technologies that could improve the competitiveness of U.S. industry and the quality of people’s lives.
This approach was summed up in a March 18, 1989 San Francisco Chronicle article, in which Shank looked forward to his directorship: “I feel I bring a view of what the industrial needs are for research, and how we can use that to have impact on the nation’s problems of competitiveness.”
Specifically, Shank believed that by focusing on long-term, precompetitive technologies, the government can help foster U.S. industry — an important boost given that many facets of the U.S. high-tech industry had slipped behind foreign competitors by the late 1980s.
Shank also took over at a time when Berkeley Lab was moving away from its flagship enterprises of basic research in physics and chemistry and toward biomedicine, materials sciences, and energy efficiency research. Indeed, the Chronicle article adds that “His appointment seems tailored to strengthen the lab’s recent focus on basic research in electronics and materials. This is a trend away from the atomic physics and accelerator technologies that made the lab famous through the 1960s.”
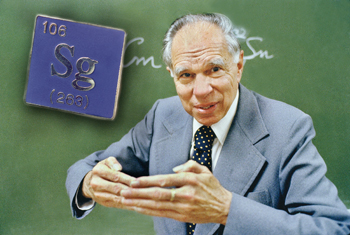
Glenn Seaborg with Seaborgium pin.
The bridge between fundamental research and research that can benefit humanity in short order was best embodied in a new facility that drew Shank’s interest from the start. The Chronicle article states that “Shank said that one ‘wonderful’ lure at Berkeley Lab is a new machine, the Advanced Light Source [ALS], due to be finished in 1993.”
The ALS, a national user facility that generates intense light for scientific research, was the world’s first third-generation synchrotron light source in its energy range. Ground was broken for the ALS, which is actually housed in the iconic dome-shaped building of Ernest Lawrence’s 184-Inch Synchrocyclotron, in 1988. Construction of the ALS was led by Jay Marx, a physicist who had directed the PEP-4 project, one of the largest particle physics experiments of its time.
“We tried to be realistic from the start about how long it would take to build the ALS and how much it would cost,” Marx once told an interviewer. He and his group succeeded magnificently, bringing the ALS project in ahead of schedule by about three days, and about $2,000 under its $100 million budget.
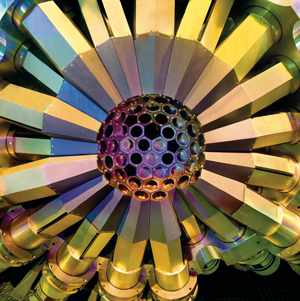
The Gammasphere.
The ALS was commissioned in March of 1993 and the official dedication took place in October, 1993. At that dedication, Shank cited six people whose contributions were critically important to the project’s completion: Jay Marx, David Attwood, Ron Yourd, Alan Jackson, Klaus Halbach, and Klaus Berkner.
By 1995, the ALS was overcommitted to users on its existing twelve beamlines, so it opened five more beamlines in 1996. That same year, to address national needs for instrumentation in macromolecular and subcellular structure determination, the ALS launched programs in materials research, chemical dynamics, and structural biology.
The opening of the ALS was big news, at least for the Lab and the scientists worldwide who lined up to use the state-of-the-art facility. But it didn’t land anybody on Jay Leno’s latenight talk show. For that, one has to find the relics of the primeval explosion that began the universe. In April, 1992, a team led by Berkeley Lab astrophysicist George Smoot did just that. These relics, the largest structures ever observed in the universe, appear as “hot” and “cold” regions with temperature differences of a hundred-thousandth of a degree.
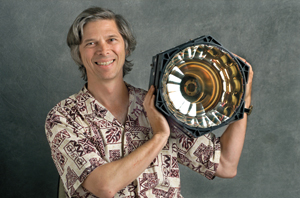
Kevin Lesko with SNO photomultiplier tube.
Smoot said the relics are believed to be the primordial seeds that grew into present-day galaxies. More than 100 million light years across, these regions were discovered after Smoot’s team analyzed data from NASA’s Cosmic Background Explorer satellite. Using this data, Smoot’s team created maps of the sky showing vast regions of space with minuscule temperature variations. The variations had been predicted by theorists but, up until Smoot and his team found evidence of them, they had never been detected.
Their discovery was splashed across headlines. It also made Smoot something of a celebrity, at least for a few days. He was a guest on Leno and he appeared in People Magazine (with Madonna on the opposite page). A more suitable and less flash-in-the-pan honor came the following year, when Smoot was named one of the winners of the 1994 E.O. Lawrence Award.
Berkeley Lab’s role as a leader in life science research was bolstered in 1992, when Lab scientists discovered a gene that predisposes people to atherosclerosis, the leading cause of heart disease in this country. Designated ATHS (for atherosclerosis susceptibility), the gene was located on chromosome 19. Berkeley Lab’s Ronald Krauss discovered the gene in collaboration with researchers from the Children’s Hospital Oakland Research Institute.
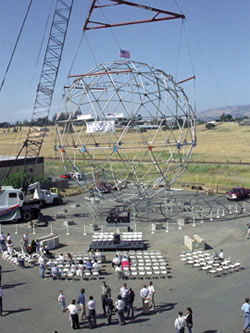
Scaffolding for the Sudbury Neutrino Observatory (SNO), Petaluma CA.
Yet another milestone came in July, 1993, when Berkeley Lab celebrated the completion of the 18-meter geodesic sphere that was to be a major component of the Sudbury Neutrino Observatory (SNO). The facility, located in a cavern more than a mile underground in Canada, is a collaboration involving institutions in the U.S., Canada, and the United Kingdom. Its mission is to study neutrinos, the ghostlike particles emitted from the sun and other cosmic and man-made sources.
Berkeley Lab’s contribution to the project was spearheaded by a team from the Nuclear Science and Engineering divisions under the leadership of physicist Kevin Lesko. The team developed the panel arrays that housed thousands of the extremely sensitive light-detectors called photomultiplier tubes. Using these arrays, SNO became the first detector sensitive enough to measure both ordinary electron neutrinos and the much rarer muon and tau neutrinos.
Also in 1994, Element 106 was named “seaborgium” in honor of Nobel Laureate and Associate Director-at-Large Glenn T. Seaborg. It was discovered at Berkeley Lab in 1974 by Lab physicist Albert Ghiorso and Lawrence Livermore National Lab chemist Kenneth Hulet, who named it in honor of Seaborg. The element was confirmed at Berkeley Lab in 1993 in an experiment at the 88-Inch Cyclotron led by Ken Gregorich and Darleane Hoffman of the Nuclear Science Division. The controversial move to name the element after the great chemist marked the first time an element had been named for a living person.
But the biggest breakthrough came on April 26, 1994, when an international collaboration of physicists, including a Berkeley Lab team, announced the first experimental evidence for the subatomic particle known as the top quark.
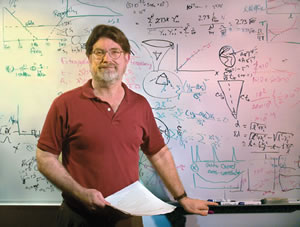
George Smoot.
The experiment was carried out at Fermi National Accelerator Lab-oratory’s Tevatron by the Collider Detector at Fermilab (CDF) collaboration. The existence of the top quark is required by the Standard Model, which holds there are six quarks that combine to form composite particles, such as protons and neutrons. The fifth of these six, called the bottom quark, was discovered at Fermilab in 1977. Scientists had been searching for its partner, the top quark, ever since.
An essential contribution to the experiment came from Berkeley Lab physicists and engineers who designed a microchip for the Silicon Vertex Detector, an extremely high resolution instrument at the heart of the CDF array that enabled precise identification and tracking of bottom quarks.
“We were confident that we would eventually find the top quark,” said Lina Galtieri in a press release on the achievement. She was a physicist who headed the CDF group at Berkeley Lab. Galtieri’s group also analyzed the CDF data to determine the mass of the top quark candidates. This analysis was based on a technique developed at Berkeley Lab in the 1960s by the research group led by the late Nobel laureate Luis Alvarez.
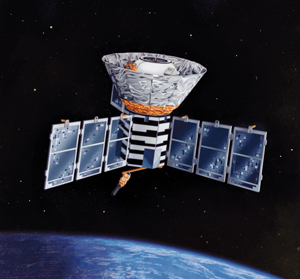
The Cosmic Microwave Background Explorer (COBE).
Importantly, the CDF group’s finding was independently corroborated by a second detector at the Tevatron called DZero, which is also a multi-institutional experiment. The DZero group at Berkeley Lab, led by Ron Madaras, was responsible for designing, building, and commissioning two critical components of the DZero detector array: the Electromagnetic End Calorimeter, which identifies and measures the energies of electrons; and the Vertex Detector, which detects the tracks left by charged particles.
In a March 2, 1995 Fermilab symposium, the CDF and DZero groups announced that they had tripled the amount of data collected since the earlier announcement, confirming the existence of the particle.
“This is one of the ten most important accomplishments in this century in this field,” said Tom Trippe at the time. Trippe is a physicist in the Physics Division and a member of the DZero group.
Berkeley Lab scored another major national user facility in November, 1995, when DOE’s Office of Energy Research announced that it had selected the Lab as the site of a new high-performance computing center. In a November, 1995 Currents article, Shank underscored the importance of the center to the future of Berkeley Lab. “We have an unprecedented opportunity to integrate science and computing to fully realize the value of high performance computing for the scientific programs of the nation,” he said. “This has the potential to transform the way we do science in virtually every part of the Laboratory.”
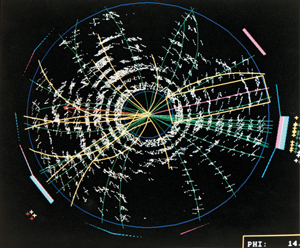
Electronic signatures revealing top quark, produced by collisions of protons and antiprotons in the Tevatron accelerator at Fermilab.
Indeed, when the center came to Berkeley Lab in May, 1996, it was named the National Energy Research Scientific Computing Center (NERSC), and forever changed the Lab’s research portfolio. Early users of NERSC’s six CRAY supercomputers included teams of scientists tackling grand challenges ranging from treating nuclear waste, understanding the human genome, studying the Big Bang, and creating the next generation of particle accelerators.
In the fall of 1995, Berkeley Lab started construction of a new Human Genome Laboratory, building 84, which was located near buildings 74 and 83 to form a cluster of life sciences research laboratories. The building brought together all of the research teams that made up Berkeley Lab’s Human Genome Center (now part of the DOE Joint Genome Institute, which was established in 1997 in Walnut Creek). The building also marked a milestone in the history of the Human Genome Project, the national effort to decipher the human genetic code spearheaded by DOE and the National Institutes of Health. Ultimately, Berkeley Lab’s genome scientists (working at the Joint Genome Institute) went on to sequence human chromosomes 5, 16, and 19, which together constitute some 11 percent of the human genome.
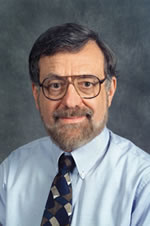
Ron Krauss.
As 1995 drew to a close, the Lab welcomed one more major research tool. On December 1, the world’s most powerful instrument for detecting gamma rays, the Gammasphere, was dedicated. The purpose of the Gammasphere was to enable scientists to observe gamma radiation emitted by rapidly spinning atomic nuclei. Such observations yield information on nuclear structure that could not be obtained from conventional studies.
Along with these changes in the Lab’s research landscape came a change in the Lab’s name. On June 16, 1995, after receiving approval from the University of California Board of Regents, the lab added the word “national” to its name, becoming Ernest Orlando Lawrence Berkeley National Laboratory, or Lawrence Berkeley National Laboratory for short. The change was made to reflect the Lab’s commitment to respond to national scientific needs, as well as to project a more distinctive identity to decision-makers in Washington. The change was also intended to distinguish the Lab from two other East Bay science facilities named after Lawrence — Lawrence Livermore National Laboratory and the Lawrence Hall of Science.